Scientific Risk Evaluations
Note: Please see physical copy of National Driller for illustrations referenced in this article.
As the demand for ground water grows, more and more water supplies are placed in close proximity to commercial and industrial facilities that may pose a risk to the safety of drinking water. Chemicals accidentally released into the ground water system may migrate into aquifers used for drinking water, thereby posing health risks. The following two case studies are examples of basic methods to determine if the water supply users were at risk from the chemical release.
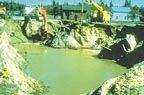
Computer Modeling
A convenience store and filling station in Virginia had three 3,000-gallon underground storage tanks (USTs) installed in 1972. The USTs were removed in 1998, at which time the tanks were discovered to have been leaking gasoline. Several community and residential water supply wells were located nearby. Four water supply wells served the convenience store and adjacent mobile home park, the closest of which was only 75 feet from the former USTs. In addition, seven private water supply wells were located within 1,000 feet of the former tank field. Several monitoring wells were installed into the shallow ground water table to collect water level data and to sample for the presence of dissolved contaminants (Figure 1).Bedrock at the site was relatively shallow, approximately 40 to 60 feet below grade. The water supply wells surrounding the site all were completed in bedrock, whereas most of the monitoring wells were completed in the shallow water table within the upper soil unit.
Water samples were collected from the site monitoring wells and the water supply wells depicted in Figure 1. The samples were analyzed for total petroleum hydrocarbons, as well as benzene, toluene, ethylbenzene, xylenes and methyl tertiary butyl ether. The results of the laboratory analysis revealed high concentrations of dissolved hydrocarbons in the shallow ground water in the former UST area. None of the residential wells on the opposite side of the street had detectable levels of contaminants.
Though the residential wells were not impacted, their proximity to the contaminated ground water was of concern. One of the first things we wanted to accomplish was to evaluate the potential for hydraulic communication between the shallow water table aquifer and the deeper bedrock aquifers. We did this by way of Well No. 2 and MW-6, located just a few feet apart at the rear of the building. We reasoned that if the shallow water table aquifer observed in MW-6 was in hydraulic communication with the bedrock aquifer in which Well No. 2 was completed, then the water level in MW-6 should respond to the pumping in Well No. 2. We started the analysis by discontinuing the pumping of Well No. 2 to allow the water level in this well to reach static conditions. When the well had been static for 36 hours, we gauged both wells, and found that the water level in MW-6 was almost 2 feet higher than that in Well No. 2. This indicated a natural downward hydraulic gradient, which would suggest a natural tendency for the shallow ground water to flow into the bedrock aquifer if hydraulic communication did exist. When pumping was resumed in Well No. 2, both wells responded immediately to the pumping as evidenced by drawdown in the wells. While the magnitude of drawdown in MW-6 was much smaller than that in Well No. 2, the immediate response suggested that hydraulic communication was taking place.
With this evidence for hydraulic communication between the contaminated shallow water table aquifer and the deeper bedrock aquifer, a computer model was constructed using the USGS code MODFLOW. The model was used to simulate the flow of ground water and the transport of dissolved hydrocarbon. Model layers were defined to mimic the upper soil zone and lower bedrock unit. The model layers were configured based on information from drilling and electrical resistivity imaging of the subsurface. Model parameters were defined based on soil properties, slug tests conducted on the monitoring wells, and the results of the pumping analysis. The water supply wells were defined in the model as pumping wells withdrawing water from the model layer in which each one was completed.
One of the basic steps in simulating ground water flow is to calibrate the model to known conditions. In this case, the model was calibrated to the observed water levels at the monitoring wells. A properly constructed and calibrated flow model should be a reasonable representation of the physical system that it is simulating. In this case, an excellent match was obtained between the simulated and observed water levels at the monitoring wells.
The calibrated flow model was then coupled with the transport model MT3D to evaluate the potential for the existing contamination to reach the water supply wells. Of particular concern was the apparent hydraulic communication between the shallow water table aquifer and the bedrock aquifer, and the fact that pumping from the water supply wells might tend to draw the contaminants to the wells.
The transport model MT3D uses the flow field from the calibrated MODFLOW model to calculate the transport of dissolved constituents under the influence of advective flow, dispersion, retardation and decay. Conservative parameters were defined for each of these processes in the interest of protecting the water supply users.
The model results indicated that numerous water supply wells to the south and east of the site could be impacted by dissolved hydrocarbons (Figure 2). Interestingly, the simulations did not indicate that Well No. 2 would be impacted by the contaminants. This was in large part because Well No. 1, constructed at a much shallower depth than Well No. 2, tended to draw the contaminants toward it and away from Well No. 2. This model provided the basis for additional simulations to design a remediation system that would adequately protect the water supply users from the existing contamination.
Cluster Analysis
A church in Virginia was discovered to have a leaking heating oil tank that had released more than 1,500 gallons of heating oil. Numerous wells were drilled into the limestone bedrock to try to find and recover the oil. An open void was discovered during the drilling, which is common in karst terrains of the area. Oil was found only in the well containing the void, raising concerns that the oil might be migrating through karst features. Of particular concern was the fact that the church and neighboring properties all were supplied by well water, and a commercial spring was located approximately 1,000 feet away in the down-gradient direction.
Computer modeling was not considered an appropriate tool to evaluate the risk to local wells and springs because of the karstic nature of the area and the scale of the problem. Moreover, water level data and contaminant distribution data suggested that the wells might be completed in more than one flow system that was distributed laterally rather than vertically. Specifically, during the January 2001 gauging event, almost 26 feet of head difference was observed between MW-5 and RW-3 over a distance of only 21 feet (Figure 3) resulting in a hydraulic gradient of 1.24 ft./ft. Moreover, the head difference between MW-1 and RW-2 was observed to be 10.04 feet during the March 2001 gauging event, resulting in a hydraulic gradient of 0.72 ft./ft between these wells. In contrast, the gradient between RW-1 and MW-7 was observed to be only 0.06 ft./ft during this same gauging event. Finally, MW-1 had been observed to contain separate phase hydrocarbon for more than a year, yet none had been observed at RW-2 only 14 feet downgradient. With more than 10 feet of head difference between them, separate phase hydrocarbon should be observed at RW-2 if these wells were experiencing free hydraulic communication between them.
The distinctness of hydrologic flow systems can be determined by evaluating differences in natural ground water chemistry. Between separate flow systems, natural differences should be observed in hydrochemical parameters such as hardness, temperature, pH, specific conductance and dissolved inorganic ions. The distinctness of the aquifers can be evaluated with statistical analyses such as multivariate cluster analysis. Cluster analysis is a multivariate statistical analysis that considers all of the input parameters and clusters the samples together according to their similarities. The result is a dendogram or tree-like diagram in which the samples most similar in their chemical makeup will join in the top branches with dissimilarity of samples and groups of samples increasing as they join down the trunk. The objective of the analysis was to use natural ground water chemistry data to evaluate the hydraulic distinctness between the ground water contaminated by the petroleum release and the ground water supplying nearby potable wells and springs.
Seven water supply wells, three springs and 11 site monitoring wells were used for this study. These wells and springs were sampled and analyzed for pH, temperature, specific conductance, hardness, bicarbonate alkalinity, carbonate alkalinity and the presence of various chemicals.
The results of the cluster analysis revealed four main clusters, two containing primarily domestic wells and two containing primarily monitoring wells (Figure 4). The monitoring wells that clustered with the domestic wells were distant from the contaminant plume and therefore did not pose a threat to those resources. However, Mace Spring clustered with several monitoring wells in Cluster #3. Not only was this spring used as a water supply, the monitoring wells in this cluster were within the contaminated ground water or in proximity to the contamination. Therefore, this spring was considered to be at risk from the dissolved contamination and a monitoring program was instituted to protect this water resource.
Looking for a reprint of this article?
From high-res PDFs to custom plaques, order your copy today!