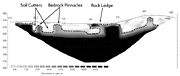



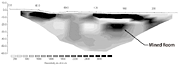

Find Drilling Targets With Electrical Resistivity Imaging
Electrical resistivity imaging is useful for finding fracture zones that are likely targets for high-yield wells. While fracture trace analysis reduces uncertainty over random drilling, electrical resistivity reduces uncertainty in that it confirms presence of a fracture zone or other drilling target. An added benefit of resistivity imaging is it finds fracture zones and other water-bearing features that have no evidence of their existence on the ground surface.
For example, Figure 6 illustrates a resistivity section from a community in the Blue Ridge province of Virginia. It is notoriously difficult to find high-yielding wells in the metamorphic rocks of the Blue Ridge. Twelve wells had previously been drilled in the community, several of which had been sited by a geologist using fracture trace analysis alone. Only six wells yielded sufficient water to bring into production, with yields ranging from 13,000 to 40,000 gallons per day (9 to 28 gpm). We conducted our own fracture trace analysis and suspected a topographic feature to be a fracture zone. We conducted electrical resistivity imaging and confirmed the topographic draw as a fracture zone as evidenced by vertical low-resistivity feature coincident with the valley. We recommended drilling on this feature, which resulted in a well yielding 114,000 gpd (79 gpm), almost three times the best yielding well located by fracture trace analysis alone.


In conclusion, this methodology combines traditional fracture trace techniques with modem geophysics. By integrating knowledge of the local geology, geomorphology and geophysics collected from the site, one may find high groundwater yields where random drilling or traditional methods alone have failed.