Innovative Methods For Locating High-Yield Water Supply Wells -- Part I
The world population is growing geometrically, projected to reach 8 - 11 billion people by 2050. Even in North America, where population growth rates are considerably lower than most of the world, the 1999 population estimate of 303 million is expected to grow to 374 million by 2050. Growing with population is the demand for increased development of water resources. Groundwater resources figure prominently throughout North America for private and public drinking water supply, irrigation, manufacturing, and mining. The United States uses approximately 76.4 billion gallons of groundwater per day for these purposes. The U.S. Geological Survey (USGS) estimates that in 1995 38 percent of the water supplied by municipal water departments in the US came from groundwater sources. Groundwater supplies served more than 97 percent of the rural population with its drinking water.
In Canada the numbers are somewhat lower, where in 1981 approximately 11 percent of municipal water was supplied by groundwater and approximately 82 percent of rural water supplies came from groundwater. This is probably due to abundance of surface water sources in Canada. Groundwater usage is expected to increase during this century as need for water increases and available sites for surface reservoirs decrease.
Ancient Methods of Finding Groundwater
One of the oldest and most controversial methods for locating groundwater is dowsing. Dowsing, also known as water-witching, divining, rhabdomancy, or doodlebugging, is locating water supplies by use of dowsing rod or pendulum. These dowsing rods can be a forked stick (Y-rod), a rod bent at a 90-degree angle (L-rod), or a straight stick (bobber). The dowser is said to be led to water supplies by movement or action of the dowsing rod. The origin of the practice is not clear, but earliest known indication of its usage occurs on a 4500 to 5000-year old grave inscription in Brittany. The first known literature reference is found in a 1540 publication on mining, De re Metallica by Georgius Agricola.
Opinions on validity of the method range from avid support to complete skepticism. Many scientific studies on the practice have yielded no concrete evidence of its validity, but many continue to use dowsers as their preferred method of locating drilling sites.
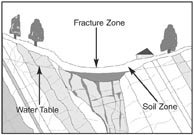
Traditional Scientific Methods
One popular method of locating drilling sites for many decades is fracture trace analysis. Particularly in bedrock aquifers, groundwater tends to be concentrated in localized fracture zones. Fracture zones are areas of higher fracture density within bedrock of lower fracture density (Figure 1). Fracture zones are generally less resistant to erosion than unfractured rock, and are more likely to form topographic low areas or stream beds.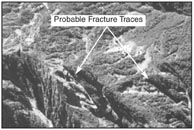

While using fracture trace analysis is an improvement over random drilling and can decrease uncertainty of obtaining high well yield, it is far from a conclusive method. At ATS we routinely become involved in water supply projects where unsuccessful wells had been drilled on features interpreted to be fracture traces. Not all linear topographic features are fracture traces, and not all fracture zones are expressed on the ground surface as linear topographic features.
Improving on Traditional Methods
Geophysical methods have been used for subsurface exploration for many years. Some of the most popular methods in recent years include seismic refraction, very low frequency radar (VLF), and electrical resistivity. Recent advances in electrical resistivity imaging make this method the most inexpensive and effective geophysical technique available.
By starting with an understanding of local geology, we conduct a fracture trace analysis to look for likely fracture zones. The final step is to apply electrical resistivity imaging to confirm or refute the existence of fracture zones.

Resistivity imaging (also called resistivity surveys, resistivity profiling, and resistivity tomography) is conducted by inducing an electric current into the ground between two electrodes, and measuring the potential at other electrodes. Numerous configurations of electrode placement are commonly employed, each with unique data characteristics. Figure 4 illustrates a schematic diagram of dipole-dipole configuration. A current is applied to two adjacent electrodes positioned a predetermined distance apart (distance a). Voltage across two other electrodes is measured simultaneously with the applied current.
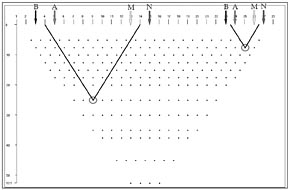
Looking for a reprint of this article?
From high-res PDFs to custom plaques, order your copy today!