Meeting the Challenges of Ground Water Sustainability
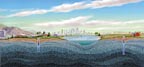
Ground water scientists have developed an expanding capability to address issues associated with the development and sustainability of ground water resources. Early efforts focused on methods of evaluating the effects of ground water pumping on an aquifer's long-term capacity to yield water to wells. Subsequently, methods were applied to evaluate various effects of ground water development on surface water bodies, land subsidence and saltwater intrusion. Starting in the late 1970s, increasing concerns about contamination of ground water by human activities led to an awareness of the great difficulty and expense of cleaning up contaminated aquifers and drew attention to the importance of prevention of ground water contamination. With time, it has become clear that the chemical, biological and physical aspects of ground water systems are interrelated and require an integrated analysis, and that many issues involving the quantity, quality and ecological aspects of surface water are interrelated with ground water. Thus, ground water hydrologists continually are challenged by the need to provide greater refinement to their analyses and to address new problems and issues as they arise.
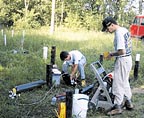
Ground Water Data
The foundation of any good ground water analysis, including those analyses with an objective to propose and evaluate alternative management strategies, is the availability of high-quality data. Some types of data commonly required, such as precipitation data, generally are available and relatively easy to obtain at the time of a hydrologic analysis. Other data and information, such as geologic and hydrogeologic maps, can require years to develop. Still other data, such as a history of water levels in different parts of ground water systems, require foresight in order to obtain measurements over time, if they are to be available at all. Thus, a key starting point for assuring a sustainable future for any ground water system is development of a comprehensive hydrogeologic data base over time. As examples, these data would include depths and thicknesses of hydrogeologic units from lithologic and geophysical well logs, water-level measurements to allow construction of predevelopment water-level maps at various times during development, ground water sampling to document pre- and post-development water quality, and simultaneous measurements of streamflow and stream quality during low flows to indicate possible contributions of discharging ground water to surface water quality. Many of the types of data and data compilations need to be viewed on maps. Thus, Geographic Information Systems (GIS) typically are an integral part of the data base system to assist in organizing, storing and displaying the substantial array of needed information.Computer Models
During the past several decades, computer simulation models for analyzing flow and solute transport in ground water and surface water systems have played an increasing role in the evaluation of alternative approaches to ground water development and management. The use of these models has somewhat paralleled advances in computing systems. Ground water models are an attempt to represent the essential features of the actual ground water system by means of a mathematical counterpart. The underlying philosophy is that an understanding of the basic laws of physics, chemistry and biology that describe ground water flow and transport, as well as an accurate description of the specific system under study, will enable a quantitative representation of the cause and effect relationships for that system. Quantitative understanding of cause and effect relationships enables forecasts to be made for any defined set of conditions. However, such forecasts, which usually are outside the range of observed conditions, typically are limited by uncertainties due to sparse and inaccurate data, poor definition of stresses acting on the system, and errors in system conceptualization. Although forecasts of future events that are based on model simulations are imprecise, they nevertheless may represent the best available decision-making information at a given time. Because of the usefulness of computer simulation for decision making, the basic construction of computer simulation models, as well as model forecasts, need to be updated periodically as the actual ground water system continues to respond to the physical and chemical stresses imposed upon on it and as new information on the ground water system becomes available.
Computer simulation models have value beyond their use as purely predictive tools. They commonly are used as learning tools to identify additional data that are required to better define and understand ground water systems. Furthermore, computer simulation models have the capability to test and quantify the consequences of various errors and uncertainties in the information necessary to determine cause and effect relationships and related model-based forecasts. This capability, particularly as it relates to forecasts, may be the most important aspect of computer models in that information about the uncertainty of model forecasts can be defined, which in turn enables water managers to evaluate the significance, and possibly unexpected consequences, of their decisions.
Strategies for Sustainability
When broadly considered, alternative management strategies are composed of a small number of general approaches, as outlined below.Use source of water other than local ground water. The main possibilities are 1) shift the source of water, either completely or in part, from ground water to surface water, or 2) import water (usually, but not necessarily, surface water) from outside river basin or ground water system boundaries.
Change rates or spatial patterns of ground water pumpage. Possibilities include 1) an increase in pumpage that results in a new equilibrium of the ground water system, 2) a decrease in pumpage that results in a new equilibrium of the ground water system, or 3) a change in the spatial distribution of pumpage to minimize its existing or potential unwanted effects. Management strategies might include varying combinations of these approaches.
Increase recharge to the ground water system. Usual options include 1) pumpage designed to induce inflow from surface water bodies, or 2) recharge of surface water or reused water (ground water or surface water) of good quality by surface spreading or injection through wells.
Decrease discharge from the ground water system. Possibilities include pumpage that is designed to decrease discharge 1) to streams, lakes or springs, or 2) from ground water evapotranspiration. Both of these possibilities can have undesirable effects on surface water bodies or on existing biological resources.
Change the volume of ground water in storage at different time scales. Possibilities include 1) managed short-term (time scale of months and years) increases and decreases in storage in the ground water reservoir, which suggest that the ground water reservoir might be managed at a time scale that is comparable to the management of surface water reservoirs, or 2) a continuing long-term (possible time scales of decades and centuries) decrease in ground water storage. Of course, complete or almost complete depletion of aquifer storage is not a strategy for sustainability, but it is instead an extreme approach that may be considered in some situations.
Consideration of these general approaches indicates that they are not mutually exclusive; that is, the various approaches overlap, or the implementation of one approach will inevitably involve or cause the implementation of another. For example, changing rates or patterns of ground water pumpage will lead to changes in spatial patterns of recharge to or discharge from ground water systems.
The short list of general approaches may suggest that proposing and evaluating alternative management strategies is deceptively simple. On the contrary, ground water is withdrawn from complex, three-dimensional systems, and many possible combinations of these approaches typically should be considered in developing management strategies for a particular ground water system.
Looking for a reprint of this article?
From high-res PDFs to custom plaques, order your copy today!