Seawater Desalination in America
Although seawater desalination has been common practice in some parts of the world for a number of years, it has historically been considered infeasible in the United States. However, the need to develop seawater desalination is upon us.
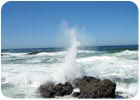
Although seawater desalination has been common practice in some parts of the world for a number of years, it has historically been considered infeasible in the United States. While there have been several small seawater desalination plants in the U.S. for number of years, most of these installations were constructed either as experimental facilities or as a result of dire projections of future water supply, despite the high cost of treatment.
For example, the City of Santa Barbara, Calif., constructed a seawater desalination plant in the mid-1990s in response to a severe drought. However, natural attenuation of the drought and high operating costs have resulted in the plant being decommissioned until future conditions require its reactivation.
Despite historical concerns over cost and concentrate disposal, however, the concept of seawater desalination using reverse osmosis (RO) membranes has evolved rapidly in the past several years from perception as an interesting but infeasible idea to a viable long-term solution for augmenting potable water supplies in populated coastal regions. Consequently, RO facilities of substantial capacity are being planned or studied by utilities serving some of America's largest population centers, as well as other growing coastal areas.
It is important to note that costs often are cited without sufficient qualifying information to allow a meaningful comparison on equal terms between the cited costs for different projects. For example, whether treated water costs are amortized over 20 or 30 years can have a significant effect on the cost, as can the assumed interest rate, neither of which are commonly cited. In addition, it may not be clear whether the cost represents that to the consumer or the overall cost without any subsidies. Moreover, water quality parameters such as average temperature and salinity may have a substantial impact on the treatment cost for seawater desalination, and this information may not be given in association with cost estimates.

In terms of capital costs, there are several factors that have contributed to the expense of seawater desalination. First, the intake and concentrate discharge structures for RO facilities generally are located in the ocean, making this infrastructure costly. This cost can be compounded by the fact that these structures often must extend a considerable distance offshore to ensure the protection of marine ecosystems and prevent the intake of extraneous material. In addition, for an RO treatment plant of substantial capacity, the size of this infrastructure may be large, further escalating the cost. For example, because the recovery of a typical RO plant is about 50 percent, the concentrate discharge line must be sized similarly to the permeate line, and intake must accommodate twice the permeate capacity. Another significant capital expense associated with RO facilities is the materials of construction that are in contact with the feed or concentrate, which not only must be able to withstand feed pressures on the order of 1,000 psi with some factor of safety, but also be resistant to corrosion in the very corrosive seawater environment. This is particularly important for the concentrate stream, which may more than have twice the TDS concentration of seawater.
Consequently, the most feasible option for seawater concentrate disposal is via ocean outfall. However, in addition to the expense of constructing an ocean outfall of substantial size, there also are significant environmental concerns. Because the introduction of the concentrate stream to the ocean at twice the ambient TDS concentration without any mitigating measures could have a significant negative impact on the local marine ecosystem, ocean discharge of seawater concentrate has been considered infeasible and extremely difficult to permit under any coastal regulatory authority. Thus, the issue of concentrate disposal has been a significant deterrent to the implementation of RO.
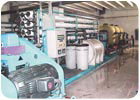
In addition, engineers have been hesitant to design or recommend these processes, particularly in the litigious climate of modern American society. Some recent examples of this are low-pressure membrane filtration (i.e., microfiltration and ultrafiltration) and ultraviolet (UV) disinfection, which utilities were wary of at first, but which subsequently generated almost unprecedented interest. Even if a certain technology has been widely implemented in other parts the world with success, there always has been a general reluctance to design the first American installation. Metaphorically speaking, sheep are plentiful, but guinea pigs are few and far between. While this industry mentality has certainly not been the primary obstacle to seawater desalination, it has been a contributing factor.
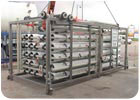
The first advantage of the co-location strategy is the use of the existing power plant ocean intake and outfall structures, thus precluding the need to construct separate such infrastructure for the RO facility and saving a substantial portion of the treatment plant capital cost. However, the most important benefit of co-location is the dilution of the RO concentrate stream prior to ocean discharge. Although the reject stream may have more than twice the concentration of TDS as the ambient seawater, the return cooling water flow may be one to two orders of magnitude greater than the concentrate flow, diluting the concentrate to near ambient levels at discharge. As a result, the environmental impact of the RO plant is minimized, facilitating the permitting process.
It is important to note, however, that the salinity of the blended discharge from a co-located RO treatment plant can vary somewhat, and is dependent on the ambient salinity, the recovery of the plant, and the ratio of the concentrate flow to the cooling water flow after withdraw of the RO facility feed. Thus, for example, if the concentrate represents a more substantial fraction of the blended flow, the salinity of the discharge may approach levels that could more significantly impact the local marine ecosystem. Consequently, environmental impacts should be carefully studied on a site-specific basis, and thorough water quality and biological monitoring should always be conducted in order to ensure the health of the marine community in the vicinity of the discharge.
Another benefit of co-location is that the heated cooling water downstream of the heat exchange process can be used as the feed for the RO facility. Because the heated water is less viscous, the pressure required to drive the feed water through the RO membranes is somewhat reduced, lowering the operating cost of the facility. Although the heated water also allows increased salt passage across the semi-permeable membrane, thus slightly reducing the rejection characteristics, the benefit of the reduced pressure requirements typically more than compensates for this minor performance loss in terms of the overall cost of the facility.
A co-located facility also may receive the benefit of a negotiated favorable power rate structure that does not include transmission charges and which facilitates the constructive use of waste energy from the power plant.
In an RO system, the pressurized feed stream (e.g., ~ 1,000 psi) enters the membrane element, where a portion of the flow passes through the semi-permeable membrane to become permeate. The remainder of the flow does not pass through the membrane, becoming increasingly concentrated along the flow path within the membrane as an increasing of amount of water is removed as permeate is produced. Although there are some losses incurred in the membrane element, most of the pressure of the feed stream is transferred to the concentrate stream as it exits the RO system. In the past, this pressure represented simply another engineering design issue, as this pressure often had to be artificially reduced in order to safely process the concentrate stream for ultimate disposal. However, the gradual development and improvement of highly efficient energy recovery devices for RO systems transformed this issue from a challenge into a process enhancement by transferring the energy associated with the pressurized concentrate stream back to the feed stream. This energy transfer allows the size of the feed pumps to be minimized, lowering power requirements and reducing energy costs substantially in some cases. Energy recovery devices typically are based on simple mechanical principles, have few moving parts, and are hydraulically driven.
Among the most significant improvements in the membrane elements is the ability to operate at lower feed pressures, while maintaining a high degree of salt rejection. The ability to operate at lower pressures reduces the life-cycle cost of the plant, since, like the energy recovery devices, this advancement allows for smaller feed pumps that require less energy to operate.
Another creative engineering approach to seawater desalination involves the use of nanofiltration (NF) membranes, which have less efficient salt rejection characteristics, but operate at lower feed pressures, in a dual-stage configuration, in which both the concentrate and the permeate associated with the first stage are further treated with a second stage of NF membranes to yield product water of drinking water quality. Pilot test results have indicated that this process can produce high quality desalinated permeate at pressures below 600 psi in the first stage and below 250 psi in the second stage, resulting in lower operating costs. The much lower pressure requirements associated with the second stage may allow the use of less expensive non-ferrous materials that are not susceptible to corrosion.
Although desalinated seawater remains more expensive than most other ground water and surface water sources in these states, the falling cost of seawater desalination and the price that water suppliers and their customers are willing to pay for badly needed new sources of supply have begun to converge. Seawater desalination also represents a drought-proof supply that can dampen the impact that dry seasons have on imported surface water. In addition, seawater desalination is a locally produced water supply with a vast source that is difficult to intentionally contaminate, factors that carry heightened importance in an age in which water security is paramount.
Moreover, more interest in seawater desalination and a favorable market will boost investment by both the public and private sectors into research dedicated to improving technology to further lower costs and/or enhance process efficiency. The value of desalinated seawater to the consumer also is an important consideration in projecting the future of the process. Even if the actual cost of seawater desalination were to stabilize, it is likely that the use of the ocean as a source of potable water would become more affordable in relative terms as a result of other water resources or uncommitted water rights become increasingly scarce. Thus, because seawater desalination is not affected by these factors, it may become more cost competitive by comparison. Another significant factor is that the ocean represents a drought-proof source, which should increase the value of desalinated seawater relative to its cost.
As the U.S. water treatment community gains more experience and familiarity with seawater desalination, more coastal utilities will study, design and construct treatment plants to tap the ocean as a potable water resource. The increase in the number of plants, in turn, will increase the demand for seawater desalination expertise, stimulating study of the process and associated technology, and resulting in a larger pool of creative talent with new and innovative ideas for optimization.
Although the market for seawater desalination almost certainly will continue to grow for the foreseeable future, there are, nevertheless, some concerns that could hinder that growth to some extent. For example, the operating costs associated with RO facilities are very sensitive to fluctuations in energy costs. Thus, if the cost of energy increases in the future (e.g., as a result of war, scarcity of supply, taxes, etc.), seawater desalination will become more expensive relative to less energy-intensive methods of generating potable water. However, this sensitivity can be moderated somewhat by advances in technology that allow membrane desalination processes to be operated at lower pressures. Another important consideration for both existing and future co-located RO treatment facility is the long-term viability of the associated power plants. If the power plant were ever to be decommissioned, the impact on the feasibility and cost of the RO facility could be sizable. For example, the water utility would be forced to continue to operate the power plant, cooling water pumps at its own cost in order to both continue to supply the treatment plant intake with feed water and to sufficiently dilute the concentrate for ocean discharge.
Because these may be large, energy intensive pumps that circulate many hundreds of millions of gallons per day, the expense of operating these pumps may be significant. In addition, even if the utility continues to operate these pumps, with the power plant out of commission, the circulated water will not undergo the heat exchange process. As a result, the RO facility will not have the advantage of a heated supply, thus increasing the viscosity of the RO membrane feed, reducing the flux and, in turn, the capacity of the treatment plant.
Another related potential complication is the finite number of suitable co-location sites, an issue compounded by the increasing difficulty of permitting new coastal power plants due to environmental concerns and the value of coastal land for other uses such real estate development, tourism and recreation. In addition, as alternative energy sources are developed, coastal fossil fuel-based power plants may become less common. Thus, as population growth in coastal areas continues largely unabated, it is important and prudent for the water treatment community to conduct ongoing research into other innovative means of facilitating seawater desalination that are not so critically dependent on power plant co-location.
Despite these concerns, ultimately it is likely that seawater desalination will eventually become commonplace in coastal areas. It is even possible at some point in the perhaps distant future that an abundance of desalinated seawater in coastal regions may help meet water demand in fast-growing interior U.S. metro areas such Atlanta, Orlando, Phoenix, Las Vegas and San Antonio, among others - either by direct piping or via coastal utilities leasing any surplus ground water or fresh surface water rights.
The relative swiftness of the water treatment community to embrace seawater desalination - going from years of entrenched belief that the process was not cost-effective or feasible to widespread study and advocacy - represents a paradigm shift of a magnitude seldom observed in the drinking water industry. One telling indicator of the acceptance of seawater desalination is that this energy-intensive process has generated the most eager enthusiasm in Southern California, a region that has some of the highest energy rates in United States.
Currently, more than 50 percent of the U.S. population lives in coastal areas, and it is anticipated that this percentage will continue to increase. Thus, with a finite supply of ground water and surface water that is reaching - and in some cases exceeding - sustainable yield, the need to develop seawater desalination will likewise continue to increase to the point at which the process is eventually transformed from a novel idea to a standard practice.

The updated roadmap is the result of three previous in which many government agency, national laboratory, university and private partners gathered to map out the future of desalination in the United States. The first roadmap identified overall goals and areas of desalination research. Brady expects the second roadmap to be completed shortly, and the Joint Water Reuse & Desalination Task Force will then submit it to Sen. Pete Domenici, chairman of the Senate Energy and Water Development Appropriations Subcommittee, Congress and, eventually, the water user and research communities. The task force consists of the Bureau of Reclamation, the WaterReuse Foundation, the American Water Works Association Research Foundation and Sandia National Laboratories.
The roadmap will recommend specific areas of potential water desalination research and development that may lead to technological solutions to water shortage problems. “Population growth in the U.S. is expected to increase 13.6 percent per decade over the next two decades,” says Hinkebein, manager of Sandia's Geochemistry Department and head of Sandia's Advanced Concepts Desalination Group. “There will be 29 percent more of us in 20 years. Put that together with an unequal distribution of people - more moving to Texas, California, Arizona and New Mexico, where fresh water is limited - and it is easy to see we are facing a challenging water future.”
Only 0.5 percent of Earth's water is directly suitable for human consumption. The rest is composed of saltwater or locked up in glaciers and icecaps. As the world's population grows, the increased water demand will have to come from someplace. Brackish water seems to be a natural source, Hinkebein says.
The roadmap will outline the specific research needed in high-impact areas to create more fresh water from currently undrinkable brackish water, from seawater and from wastewater. It will ensure that different organizations are not duplicating research.
Water desalination is not a new concept. In the United States, the largest plants are in El Paso and Tampa. It also is commonplace in other parts of the world. Except for the Middle East, most desalination is done through reverse osmosis.
Brady says 43 research areas have been tentatively identified and some projects are already under way, jump-started with $2 million made available for the preliminary research through a matching grant from the California Department of Water Resources. California provided $1 million and members of the Joint Water Reuse and Desalination Task Force each contributed $250,000. Another $4 million in fiscal years 2004, 2005 and 2006 through federal Energy and Water Development Appropriations bills secured by Domenici also has funded desalination research at Sandia.
“The task force will decide which of the 43 projects get to the top of the research pile,” Brady explains. “As more money is made available, universities, research groups, national laboratories and private companies will bid on projects.”
Among the 43 research areas included in the roadmap:
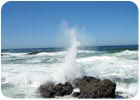
The biggest impediment to seawater desalination in this country has been the expense of treatment.
Although seawater desalination has been common practice in some parts of the world for a number of years, it has historically been considered infeasible in the United States. While there have been several small seawater desalination plants in the U.S. for number of years, most of these installations were constructed either as experimental facilities or as a result of dire projections of future water supply, despite the high cost of treatment.
For example, the City of Santa Barbara, Calif., constructed a seawater desalination plant in the mid-1990s in response to a severe drought. However, natural attenuation of the drought and high operating costs have resulted in the plant being decommissioned until future conditions require its reactivation.
Despite historical concerns over cost and concentrate disposal, however, the concept of seawater desalination using reverse osmosis (RO) membranes has evolved rapidly in the past several years from perception as an interesting but infeasible idea to a viable long-term solution for augmenting potable water supplies in populated coastal regions. Consequently, RO facilities of substantial capacity are being planned or studied by utilities serving some of America's largest population centers, as well as other growing coastal areas.
It is important to note that costs often are cited without sufficient qualifying information to allow a meaningful comparison on equal terms between the cited costs for different projects. For example, whether treated water costs are amortized over 20 or 30 years can have a significant effect on the cost, as can the assumed interest rate, neither of which are commonly cited. In addition, it may not be clear whether the cost represents that to the consumer or the overall cost without any subsidies. Moreover, water quality parameters such as average temperature and salinity may have a substantial impact on the treatment cost for seawater desalination, and this information may not be given in association with cost estimates.

Two innovations that have had significant impacts are pressure recovery devices and improved membrane elements. Photo courtesy of Bushnak Water Group.
Cost of Treatment
The high cost of seawater desalination has been the single most significant factor limiting the use of the ocean as a vast potable water resource. Although both the capital and operating costs of seawater desalination have prohibitive aspects, the greatest contributing component is the substantial energy requirements. The RO process is predicated on the application of pressure to force water through a semi-permeable membrane against the natural osmotic gradient, resulting in a permeate (or product) stream very low in total dissolved solids (TDS) and a concentrate (or reject) stream of brine very high in TDS. Because the osmotic gradient increases with TDS, a source water with an extremely high concentration of dissolved solids, such as seawater, necessitates higher feed pressure to overcome this natural gradient. In general, pressures in the range of about 1,000 psi must be continually applied to desalinate seawater using a single pass RO process, requiring the use of powerful and energy-intensive feed pumps. Higher energy requirements (and therefore higher costs) typically are associated with colder, more saline water, while less energy is required to treat warmer water with lower TDS.In terms of capital costs, there are several factors that have contributed to the expense of seawater desalination. First, the intake and concentrate discharge structures for RO facilities generally are located in the ocean, making this infrastructure costly. This cost can be compounded by the fact that these structures often must extend a considerable distance offshore to ensure the protection of marine ecosystems and prevent the intake of extraneous material. In addition, for an RO treatment plant of substantial capacity, the size of this infrastructure may be large, further escalating the cost. For example, because the recovery of a typical RO plant is about 50 percent, the concentrate discharge line must be sized similarly to the permeate line, and intake must accommodate twice the permeate capacity. Another significant capital expense associated with RO facilities is the materials of construction that are in contact with the feed or concentrate, which not only must be able to withstand feed pressures on the order of 1,000 psi with some factor of safety, but also be resistant to corrosion in the very corrosive seawater environment. This is particularly important for the concentrate stream, which may more than have twice the TDS concentration of seawater.
Concentrate Disposal
In addition to the high cost, another significant obstacle to more widespread implementation of seawater desalination is the problematic issue of concentrate disposal. The issue of concentrate disposal has become one of the biggest concerns associated with brackish ground water desalination, and this issue is compounded for the desalination of seawater sources, which generates a reject stream that may have many times the TDS concentration of brackish water reject. As a result of this very high TDS, many of the options potentially available for the disposal of brackish water reject may not be feasible for seawater concentrate, such as discharge to the sanitary sewer or a fresh surface water body, land application or deep well injection.Consequently, the most feasible option for seawater concentrate disposal is via ocean outfall. However, in addition to the expense of constructing an ocean outfall of substantial size, there also are significant environmental concerns. Because the introduction of the concentrate stream to the ocean at twice the ambient TDS concentration without any mitigating measures could have a significant negative impact on the local marine ecosystem, ocean discharge of seawater concentrate has been considered infeasible and extremely difficult to permit under any coastal regulatory authority. Thus, the issue of concentrate disposal has been a significant deterrent to the implementation of RO.
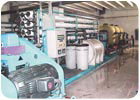
A reverse osmosis desalination plant at a resort hotel. Photo courtesy of Specific Equipment Co.
Industry Conservatism
A less tangible impediment to seawater desalination in the United States is historical conservatism of the American water treatment community. New and innovative treatment technology or ideas may be the subject of frequent study and experimentation, but these same innovations seldom are employed at full-scale either widely or rapidly. Although this conservatism may be appropriate to ensure the protection of public health through known and well-established treatment techniques, it also results in a slow pace of change in adopting some practices that may ultimately be demonstrated to be practical, effective and efficient. While many utilities throughout the U.S. are progressive, relatively few have a revolutionary mindset and thus are disinclined to build the first installation using a technology or process that may represent the leading edge of a paradigm shift.In addition, engineers have been hesitant to design or recommend these processes, particularly in the litigious climate of modern American society. Some recent examples of this are low-pressure membrane filtration (i.e., microfiltration and ultrafiltration) and ultraviolet (UV) disinfection, which utilities were wary of at first, but which subsequently generated almost unprecedented interest. Even if a certain technology has been widely implemented in other parts the world with success, there always has been a general reluctance to design the first American installation. Metaphorically speaking, sheep are plentiful, but guinea pigs are few and far between. While this industry mentality has certainly not been the primary obstacle to seawater desalination, it has been a contributing factor.
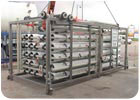
One million cubic meter desalination plant, specifically designed to work in extreme conditions. Photo courtesy of Watermatic.
Sufficient Water Resources
Another factor that has inhibited seawater desalination in the U.S. is the historical availability of a plentiful supply of fresh surface water and ground water resources. The issues associated with cost, concentrate disposal and water treatment community conservatism may have been more readily overcome if the demand for new water sources had been more severe in the past. While water supply has long been an important and pressing issue in the U.S., the availability of other sources at lower cost has precluded the need for seawater desalination. In addition, because the cost of seawater desalination has been so high by comparison, it historically has been cheaper to resort to what, at least superficially, seem like more extreme and unorthodox measures when the demand for new water resources has become critical, such as wastewater reuse and building complex infrastructure to divert water from places of abundance to those of greatest need over hundreds of miles. As a result, the demand for seawater desalination has not been prevalent.Contemporary Strategies and the Latest Solutions
Perhaps the most significant innovation that has enabled seawater desalination to become a viable process in the U.S. is the idea of co-locating RO facilities with existing water-cooled coastal power plants, a strategy which offers a number of important benefits and directly addresses some of the most critical historical hindrances. Many of the RO facilities under development or consideration in the U.S. at this time utilize co-location, which involves siting the treatment plant adjacent to a power plant, and tapping the cooling water line for the treatment plant intake and concentrate discharge. In general, this cooling water is drawn directly from the ocean in quantities ranging from several hundred million to more than a billion gallons per day (far eclipsing the flows associated with a typical water treatment plant) and is subsequently discharged back into the ocean, altered only in terms of increased temperature. In turn, a co-located RO plant draws heated seawater from the cooling water loop for its feed supply and then discharges the concentrate stream back into the cooling water loop at a point downstream of the feed withdrawal. In essence, the RO facility “piggybacks” the cooling water loop, withdrawing a small portion of the flow for feed and discharging approximately half that flow back into the loop as concentrate (assuming recovery of about 50 percent).The first advantage of the co-location strategy is the use of the existing power plant ocean intake and outfall structures, thus precluding the need to construct separate such infrastructure for the RO facility and saving a substantial portion of the treatment plant capital cost. However, the most important benefit of co-location is the dilution of the RO concentrate stream prior to ocean discharge. Although the reject stream may have more than twice the concentration of TDS as the ambient seawater, the return cooling water flow may be one to two orders of magnitude greater than the concentrate flow, diluting the concentrate to near ambient levels at discharge. As a result, the environmental impact of the RO plant is minimized, facilitating the permitting process.
It is important to note, however, that the salinity of the blended discharge from a co-located RO treatment plant can vary somewhat, and is dependent on the ambient salinity, the recovery of the plant, and the ratio of the concentrate flow to the cooling water flow after withdraw of the RO facility feed. Thus, for example, if the concentrate represents a more substantial fraction of the blended flow, the salinity of the discharge may approach levels that could more significantly impact the local marine ecosystem. Consequently, environmental impacts should be carefully studied on a site-specific basis, and thorough water quality and biological monitoring should always be conducted in order to ensure the health of the marine community in the vicinity of the discharge.
Another benefit of co-location is that the heated cooling water downstream of the heat exchange process can be used as the feed for the RO facility. Because the heated water is less viscous, the pressure required to drive the feed water through the RO membranes is somewhat reduced, lowering the operating cost of the facility. Although the heated water also allows increased salt passage across the semi-permeable membrane, thus slightly reducing the rejection characteristics, the benefit of the reduced pressure requirements typically more than compensates for this minor performance loss in terms of the overall cost of the facility.
A co-located facility also may receive the benefit of a negotiated favorable power rate structure that does not include transmission charges and which facilitates the constructive use of waste energy from the power plant.
Technological Advancements
Advances in technology also have played an important role in the recent surge of interest in seawater desalination. Although a number of innovations have contributed in small ways, the two that have had the most significant impact are pressure recovery devices and improved membrane elements.In an RO system, the pressurized feed stream (e.g., ~ 1,000 psi) enters the membrane element, where a portion of the flow passes through the semi-permeable membrane to become permeate. The remainder of the flow does not pass through the membrane, becoming increasingly concentrated along the flow path within the membrane as an increasing of amount of water is removed as permeate is produced. Although there are some losses incurred in the membrane element, most of the pressure of the feed stream is transferred to the concentrate stream as it exits the RO system. In the past, this pressure represented simply another engineering design issue, as this pressure often had to be artificially reduced in order to safely process the concentrate stream for ultimate disposal. However, the gradual development and improvement of highly efficient energy recovery devices for RO systems transformed this issue from a challenge into a process enhancement by transferring the energy associated with the pressurized concentrate stream back to the feed stream. This energy transfer allows the size of the feed pumps to be minimized, lowering power requirements and reducing energy costs substantially in some cases. Energy recovery devices typically are based on simple mechanical principles, have few moving parts, and are hydraulically driven.
Among the most significant improvements in the membrane elements is the ability to operate at lower feed pressures, while maintaining a high degree of salt rejection. The ability to operate at lower pressures reduces the life-cycle cost of the plant, since, like the energy recovery devices, this advancement allows for smaller feed pumps that require less energy to operate.
Engineering Solutions
Creative engineering solutions also have contributed to the increased viability of seawater desalination. For example, where possible, RO facilities are being sited in less saline bays that are fed by significant fresh surface water runoff. Because the salinity affects the required feed pressure, a less saline source allows for operation at lower pressures, reducing energy costs. In addition, higher recoveries also are possible with lower source water salinity, reducing the size the treatment plant and lowering the capital cost requirements.Another creative engineering approach to seawater desalination involves the use of nanofiltration (NF) membranes, which have less efficient salt rejection characteristics, but operate at lower feed pressures, in a dual-stage configuration, in which both the concentrate and the permeate associated with the first stage are further treated with a second stage of NF membranes to yield product water of drinking water quality. Pilot test results have indicated that this process can produce high quality desalinated permeate at pressures below 600 psi in the first stage and below 250 psi in the second stage, resulting in lower operating costs. The much lower pressure requirements associated with the second stage may allow the use of less expensive non-ferrous materials that are not susceptible to corrosion.
Improved and Creative Financing
Another critical factor in the increased feasibility of seawater desalination plants in the U.S. is improved and creative financing strategies. One such strategy that has garnered widespread interest is the use of modern contracting arrangements, such as design-build and its variants, including design-build-operate, design-build-own-operate and design-build-own-operate-transfer. These contract structures can reduce capital and/or operating costs, making seawater desalination facilities more affordable. As the name suggests, the design-build process allows a single contractor to both design and build the plant, reducing the number of independent parties involved and the commensurate overhead and profit. The own-operate-transfer variants also incorporate some degree of privatization, which can lower operating costs. Other financing strategies include extending the amortization period to spread the capital cost over a longer period of time and issuing bonds to generate revenue for facility construction. Utilities may be able to secure and issue bonds at lower rates than are otherwise obtainable on the open market, helping to minimize financing costs.Additional Water Resources
The increasing population of the warm and arid American Southwest, as well as the East, West and Gulf coasts of the U.S. increasingly has stressed available ground water and fresh surface water sources in recent years. Even with measures such as conservation and wastewater reuse, the need for additional water resources continually is increasing. Accordingly, the most significant interest in seawater desalination has been generated in warm coastal states such as California, Texas and Florida, three of the four most populous states in America. In Florida, where most the potable water comes from ground water sources, there are serious concerns that the rate of population growth and commensurate water demand will outpace the aquifer recharge rate in the near future. Like Florida, Texas relies significantly on ground water to meet its water supply needs, and must develop alternative sources to help satisfy future demand. Southern California is home to a rapidly growing population of approximately 20 million, and must plan to accommodate a gradual reduction in its allotment of water from the Colorado River - one of the major potable water sources for the region - as a result of neighboring states claiming a greater portion of their historic entitlement. Even areas along the northern California coast are considering long-term options for meeting the increasing water demand of a growing population.Although desalinated seawater remains more expensive than most other ground water and surface water sources in these states, the falling cost of seawater desalination and the price that water suppliers and their customers are willing to pay for badly needed new sources of supply have begun to converge. Seawater desalination also represents a drought-proof supply that can dampen the impact that dry seasons have on imported surface water. In addition, seawater desalination is a locally produced water supply with a vast source that is difficult to intentionally contaminate, factors that carry heightened importance in an age in which water security is paramount.
Future Projections
As interest in seawater desalination continues to grow, the factors that have enabled seawater desalination to become more feasible in the U.S. should create increasingly favorable conditions for the process to flourish. With more RO facilities moving from the studying or planning phase into construction, costs should continue to decline as a result of economies of scale. In addition, an increase in the number of RO facilities also will stimulate increased competition among existing equipment suppliers and encourage new suppliers to enter the market, both of which will reduce equipment costs somewhat. Over time, overall cost reductions should increase the number of feasible sites for co-location with coastal water-cooled power plants, as sites that currently may be undesirable as a result other cost-prohibitive constraints may become more economical.Moreover, more interest in seawater desalination and a favorable market will boost investment by both the public and private sectors into research dedicated to improving technology to further lower costs and/or enhance process efficiency. The value of desalinated seawater to the consumer also is an important consideration in projecting the future of the process. Even if the actual cost of seawater desalination were to stabilize, it is likely that the use of the ocean as a source of potable water would become more affordable in relative terms as a result of other water resources or uncommitted water rights become increasingly scarce. Thus, because seawater desalination is not affected by these factors, it may become more cost competitive by comparison. Another significant factor is that the ocean represents a drought-proof source, which should increase the value of desalinated seawater relative to its cost.
As the U.S. water treatment community gains more experience and familiarity with seawater desalination, more coastal utilities will study, design and construct treatment plants to tap the ocean as a potable water resource. The increase in the number of plants, in turn, will increase the demand for seawater desalination expertise, stimulating study of the process and associated technology, and resulting in a larger pool of creative talent with new and innovative ideas for optimization.
Although the market for seawater desalination almost certainly will continue to grow for the foreseeable future, there are, nevertheless, some concerns that could hinder that growth to some extent. For example, the operating costs associated with RO facilities are very sensitive to fluctuations in energy costs. Thus, if the cost of energy increases in the future (e.g., as a result of war, scarcity of supply, taxes, etc.), seawater desalination will become more expensive relative to less energy-intensive methods of generating potable water. However, this sensitivity can be moderated somewhat by advances in technology that allow membrane desalination processes to be operated at lower pressures. Another important consideration for both existing and future co-located RO treatment facility is the long-term viability of the associated power plants. If the power plant were ever to be decommissioned, the impact on the feasibility and cost of the RO facility could be sizable. For example, the water utility would be forced to continue to operate the power plant, cooling water pumps at its own cost in order to both continue to supply the treatment plant intake with feed water and to sufficiently dilute the concentrate for ocean discharge.
Because these may be large, energy intensive pumps that circulate many hundreds of millions of gallons per day, the expense of operating these pumps may be significant. In addition, even if the utility continues to operate these pumps, with the power plant out of commission, the circulated water will not undergo the heat exchange process. As a result, the RO facility will not have the advantage of a heated supply, thus increasing the viscosity of the RO membrane feed, reducing the flux and, in turn, the capacity of the treatment plant.
Another related potential complication is the finite number of suitable co-location sites, an issue compounded by the increasing difficulty of permitting new coastal power plants due to environmental concerns and the value of coastal land for other uses such real estate development, tourism and recreation. In addition, as alternative energy sources are developed, coastal fossil fuel-based power plants may become less common. Thus, as population growth in coastal areas continues largely unabated, it is important and prudent for the water treatment community to conduct ongoing research into other innovative means of facilitating seawater desalination that are not so critically dependent on power plant co-location.
Despite these concerns, ultimately it is likely that seawater desalination will eventually become commonplace in coastal areas. It is even possible at some point in the perhaps distant future that an abundance of desalinated seawater in coastal regions may help meet water demand in fast-growing interior U.S. metro areas such Atlanta, Orlando, Phoenix, Las Vegas and San Antonio, among others - either by direct piping or via coastal utilities leasing any surplus ground water or fresh surface water rights.
Summary and Conclusion
Despite a number of historical limitations, including the high cost of treatment, concentrate disposal issues and industry conservatism, seawater desalination has rapidly become a topic of serious consideration for augmenting precious potable water supplies in populous and rapidly growing coastal areas. While these limitations remain major concerns, a number of significant developments in recent years have made seawater desalination much more feasible and cost-competitive with other potential options for developing new water resources.The relative swiftness of the water treatment community to embrace seawater desalination - going from years of entrenched belief that the process was not cost-effective or feasible to widespread study and advocacy - represents a paradigm shift of a magnitude seldom observed in the drinking water industry. One telling indicator of the acceptance of seawater desalination is that this energy-intensive process has generated the most eager enthusiasm in Southern California, a region that has some of the highest energy rates in United States.
Currently, more than 50 percent of the U.S. population lives in coastal areas, and it is anticipated that this percentage will continue to increase. Thus, with a finite supply of ground water and surface water that is reaching - and in some cases exceeding - sustainable yield, the need to develop seawater desalination will likewise continue to increase to the point at which the process is eventually transformed from a novel idea to a standard practice.

Sandia researchers Michael Hibbs (foreground) and Chris Cornelius check out an electrodialysis system that removes salt from water with an electric field and special ion-exchange membranes. Photo by Randy Montoya.
Sidebar: Desalination Research Roadmap
After one last meeting this past spring, Sandia National Laboratories researchers Pat Brady and Tom Hinkebein are putting the final touches on the updated Desalination and Water Purification Roadmap that should result in more fresh water in parts of the world where potable water is scarce.The updated roadmap is the result of three previous in which many government agency, national laboratory, university and private partners gathered to map out the future of desalination in the United States. The first roadmap identified overall goals and areas of desalination research. Brady expects the second roadmap to be completed shortly, and the Joint Water Reuse & Desalination Task Force will then submit it to Sen. Pete Domenici, chairman of the Senate Energy and Water Development Appropriations Subcommittee, Congress and, eventually, the water user and research communities. The task force consists of the Bureau of Reclamation, the WaterReuse Foundation, the American Water Works Association Research Foundation and Sandia National Laboratories.
The roadmap will recommend specific areas of potential water desalination research and development that may lead to technological solutions to water shortage problems. “Population growth in the U.S. is expected to increase 13.6 percent per decade over the next two decades,” says Hinkebein, manager of Sandia's Geochemistry Department and head of Sandia's Advanced Concepts Desalination Group. “There will be 29 percent more of us in 20 years. Put that together with an unequal distribution of people - more moving to Texas, California, Arizona and New Mexico, where fresh water is limited - and it is easy to see we are facing a challenging water future.”
Only 0.5 percent of Earth's water is directly suitable for human consumption. The rest is composed of saltwater or locked up in glaciers and icecaps. As the world's population grows, the increased water demand will have to come from someplace. Brackish water seems to be a natural source, Hinkebein says.
The roadmap will outline the specific research needed in high-impact areas to create more fresh water from currently undrinkable brackish water, from seawater and from wastewater. It will ensure that different organizations are not duplicating research.
Water desalination is not a new concept. In the United States, the largest plants are in El Paso and Tampa. It also is commonplace in other parts of the world. Except for the Middle East, most desalination is done through reverse osmosis.
Brady says 43 research areas have been tentatively identified and some projects are already under way, jump-started with $2 million made available for the preliminary research through a matching grant from the California Department of Water Resources. California provided $1 million and members of the Joint Water Reuse and Desalination Task Force each contributed $250,000. Another $4 million in fiscal years 2004, 2005 and 2006 through federal Energy and Water Development Appropriations bills secured by Domenici also has funded desalination research at Sandia.
“The task force will decide which of the 43 projects get to the top of the research pile,” Brady explains. “As more money is made available, universities, research groups, national laboratories and private companies will bid on projects.”
Among the 43 research areas included in the roadmap:
- Membrane technologies (mainly reverse osmosis) that desalinate and purify water by pushing it through a semi-permeable membrane that removes contaminants.
- Alternative technologies that take advantage of nontraditional methods.
- Concentrate management technologies that consider the disposal and/or beneficial use of desalination waste streams.
- Reuse/recycling technologies that look at ways membrane and alternative technologies can be used to more efficiently recycle water.
Looking for a reprint of this article?
From high-res PDFs to custom plaques, order your copy today!