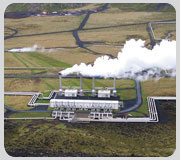
Factors affecting the cost of geothermal power development and production often are poorly understood by persons not directly involved in geothermal project development.
Drilling a geothermal well consists of a succession of steps of drilling and well casing construction until the top of the resource is reached. Once the well penetrates the geothermal reservoir, the only additional casing that may be required would be an un-cemented slotted liner to prevent rocks and debris from falling into the wellbore. However, if the formation rock is competent, then no additional casing is required. The productivity of the well will be influenced by its length in the permeable rock, as well as the number of productive fractures it crosses. Drilling costs are highly dependent upon resource characteristics. Other economic parameters may, however, also influence the total cost of drilling.
The depth of the resource is one of the major parameters influencing the cost of drilling a geothermal well. Along with the rock formation (nature, structure and hardness), which determines the drilling speed, these parameters influence the initial well diameter, the number of casing strings needed and, thereby, the time required to drill the well. According to the variability of these parameters, the drilling of a geothermal well may last from 25 days to more than 90 days, with a reasonable average of 45 days. Deeper wells also require larger - and thus more expensive - drilling rigs.
The pressure of the geothermal resource and rock formations above it will influence both the drilling process and the strength of the well casing. High pressure may result in well blowout. In addition to being dangerous for the drilling crew, well blowout may be very expensive and can threaten the economic viability of the project. In order to prevent this, the developer must install a stronger and heavier casing along with a highly specialized blowout prevention system. This may involve the drilling of a larger, and thus more expensive, well diameter, the handling of a specialized well casing and the use of special and better quality blowout prevention equipment.
When the geological formation above the resource has an internal pressure less than hydrostatic and is permeable, loss of circulation fluid may become problematic. In such cases, the drilling process may require more frequent casing stages or other specific measures (e.g., use of lower density drilling fluids) that significantly increase drilling costs. Each additional casing layer results in additional work (casing construction and well head completion), delays the drilling process (drilling activities are on hold during these casing and wellhead completion activities), and results in a narrower well diameter (which will affect the brine flow and, thereby, the well productivity). In some cases, compressed air, aerated mud or water as well as foam can be utilized as a drilling media rather than drilling mud. Compressed air or similar systems increase the cost of drilling for both the equipment that compresses the air and the system to handle the exhausted air and cuttings discharged from the well.
Loss of circulation fluid in a productive zone of the resource has a major effect on drilling costs. If the drill bit enters a highly permeable zone (e.g., a open fracture) in which formation pressure doesn't compensate for the pressure of the column of drilling fluids, this fluid may flow into the resource rock formation (i.e., loss of circulation fluid). If the developer wants to pursue the drilling to enhance the productivity of the well, that zone must be temporarily plugged with specific material (e.g., cotton seed hulls). This decision is difficult to make, however, because the productive zone may remain permanently plugged.
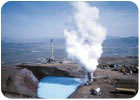
Drilling costs are highly dependent upon resource characteristics.
The chemistry of the brine is another important factor that determines the nature of the materials used in the well casing process. A corrosive geo-thermal fluid may require the use of resistant pipes and cement. Adding a titanium liner to protect the casing may significantly increase the cost of a well. This kind of requirement is rare, and in the United States, is limited to the Salton Sea geothermal resource in Southern California.
Statistical analyses of historical drilling costs data show that the depth of the well is the major parameter explaining its overall cost. It is estimated that 56 percent of the cost variability of geothermal wells is linked to depth. The diameter of the well is another important parameter influencing the cost of drilling.
More generally, drilling costs vary with the operating time of the drilling rig. Since this time may vary widely, the resulting cost of individual wells fluctuate accordingly. Inexpensive wells may cost as little as $1 million, while expensive wells may cost over $8 million to $9 million. An average well-cost estimate probably would be in the range of $2 million to $5 million. Inexpensive wells usually correspond to shallow resources located in sedimentary rock areas while expensive wells usually are characterized by deep reservoirs located in hard rock formations with corrosive brine and/or those that are under high pressure.
This article is provided through the courtesy of the Geothermal Energy Association. It is excerpted from the report, “Factors Affecting the Costs of Geothermal Power Development,” prepared by Cedric Hance, for the U.S. Department of Energy.

This 3D model of a geothermal power plant was created by Steve Anderson of Power Engineers Inc., Hailey, Idaho.
Sidebar: Geothermal Power Poised to Double
A new survey shows a major surge in developing geothermal power projects in the United States. Some 45 projects are underway that could nearly double U.S. geothermal power output according to the Geothermal Energy Association (GEA).The survey identified new power projects in Alaska, Arizona, California, Hawaii, Idaho, New Mexico, Nevada, Oregon and Utah. These projects, when developed, would provide between 1,817.9 MW and 2094.9 MW of new electric power for the grid. This would be enough electricity to meet the needs of cities the size of Albuquerque, Las Vegas, Sacramento and Seattle combined, GEA notes.
“New federal and state initiatives to promote geothermal energy are paying off,” comments Karl Gawell, GEA's executive director. “State renewable standards, coupled with the federal production tax credit, are creating a renaissance in U.S. geothermal power production,” he adds.
According to Gawell, the most significant catalyst behind this new industry activity was passage of the Energy Policy Act by Congress (EPAct) in 2005. EPAct made new geothermal plants eligible for the full federal production tax credit, previously available only to wind projects. It also authorized and directed increased funding for research by the Department of Energy (DOE), and gave the Bureau of Land Management (BLM) new legal guidance and secure funding to address its backlog of geothermal leases and permits, according to GEA.
“If we can build and sustain the momentum that EPAct has given the industry, geothermal energy can become a major U.S. energy source. But we face serious challenges this year in Congress,” Gawell warns.
The FY 2007 Budget could undermine several of EPAct's initiatives and be a major set-back to the industry's progress, GEA asserts. “Instead of increasing DOE funding, the budget proposes to zero-out geothermal research. Instead of providing the resources needed for BLM to work off its 25-year backlog of lease applications, the budget proposes to repeal this and other provisions of EPAct. Finally, the budget is silent on extending the production tax credit beyond its current deadline of December 31, 2007, a date most of these new projects will not be able to meet,” according to Gawell.